Paleomodelling using MPI-ESM
What causes abrupt climate changes during the last deglaciation?
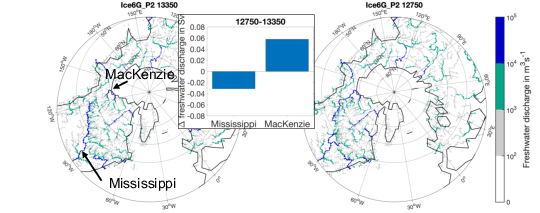
Deglacial simulations with a fully coupled atmosphere-ocean-ice sheet solid earth model confirm that the last deglaciation is not continuous and gradual but characterized by periods of massive iceberg/freshwater input into the North Atlantic. In these simulations abrupt cooling events occur due to changes in the North Atlantic overturning circulation caused by ice sheet surges and meltwater release from the decaying northern hemisphere ice sheets.
The decay of the ice sheets during the last deglaciation is a highly dynamic process and periods of abrupt climate changes are associated with massive iceberg/freshwater input into the North Atlantic. The consequence of freshwater input is a reduction in the AMOC and meridional heat transport, leading to a strong cooling in the northern North Atlantic and an extended sea ice cover. Another mechanism that leads to a sudden cooling over the North Atlantic and changes in the AMOC is associated with the redirection of meltwater from the Laurentide ice sheet (Fig. Y). During the early deglaciation most of the meltwater is discharged through the Mississippi river into the Gulf of Mexico. The retreat of the ice sheet and the accompanying changes in the ice-sheet extent allow for a redirection of meltwater through the St. Lawrence river into the northwest Atlantic and/or the Mackenzie river into the Arctic. Freshwater release into these areas leads to a sudden weakening of the AMOC and North Atlantic cooling.
Do deglacial climate changes affect the tropics?
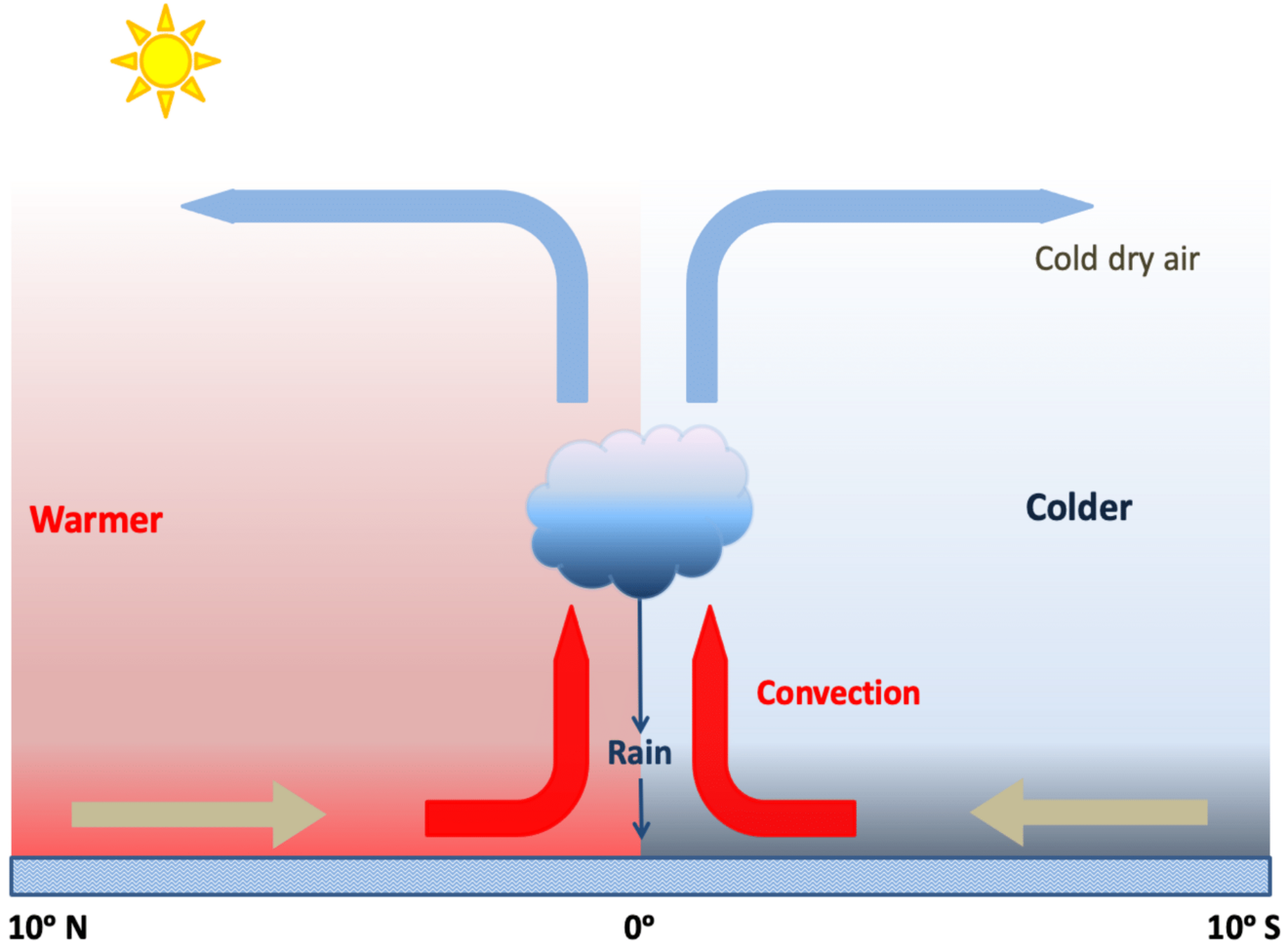
During the deglacial, the tropical rain belts shift northward; monsoons intensify and expand spatially. The abrupt climate fluctuations in the deglacial also produce corresponding oscillations in monsoon intensity and northward extent. Here, we use a diagnostic tool to analyze these processes and their interrelations.
The melting of the ice sheets, mainly in the northern hemisphere, produces differential warming between the hemispheres. This leads to a northward shift of the ITCZ (interhemispheric convergence zone). Even though the most dramatic climate change during the deglacial is in the high northern latitudes, the rising CO2 causes global warming. The amount of water vapor in the atmosphere increases. Water vapor affects monsoon precipitation in three different ways:
-
It is a potent greenhouse gas and hence can increase local surface temperature.
-
It enhances the energy of the lower atmosphere.
-
For the same monsoon winds, an increase in water vapor increases the amount of moisture flowing into the monsoon domain.
An emerging theory of monsoons based on the energy exported allows us to determine which effect of water vapor drives the deglacial changes in monsoons. We have used this theory to diagnose the transient simulation of the deglacial in the MPI-ESM.
The abrupt climate events during the deglacial modulate the inter-hemispheric temperature difference. Thus, the latitude of the ITCZ shifts. The monsoon strength is also affected due to changes in the vertical stability of the atmosphere.
The biogeochemical implications of deglacial climate change in the Mediterranean Sea
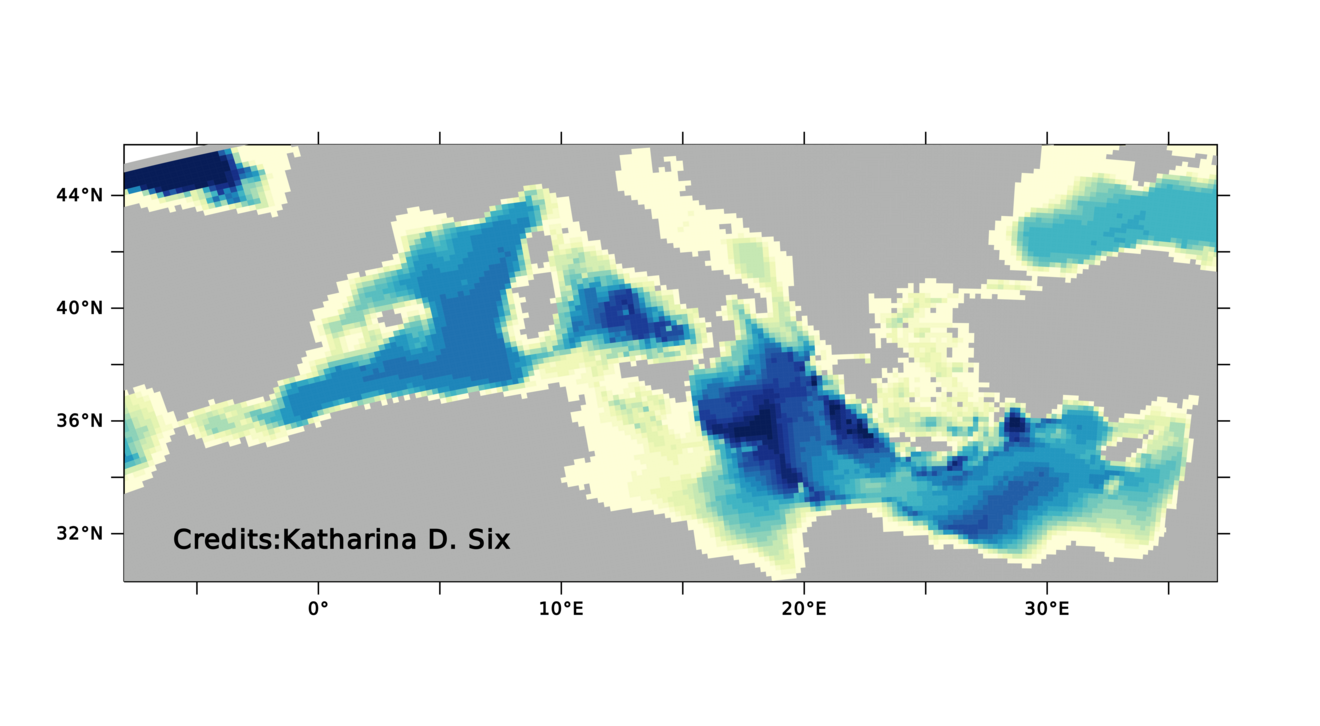
Compared to the present day, lower biological surface production, but higher sediment deposition rates are found during the Last Glacial Maximum. Temperature, circulation, sea level change and topography have an imapct on the biogeochemical patterns of the past.
As the global ocean, marginal seas such as the semi-enclosed Mediterranean Sea were subject to pronounced changes during the deglaciation. For example, the thermohaline-driven water mass exchange with the North Atlantic was slowed down and additionally modulated by a shallower sill depth at the Strait of Gibraltar due to a lower global sea level during the Last Glacial Maximum (LGM). Organic sediments that accumulate on the ocean floor preserve a long-term history of climate information. They are like a window into paleooceanography and the past marine biogeochemical cycles. However, the prevailing biogeochemical conditions from the LGM to the Holocene and the underlying mechanisms cannot be inferred from sediment data alone.
We apply a regional ocean-biogeochemical model of the Mediterranean Sea to address first the impact of altered LGM conditions on the biogeochemical realm. We find significant higher deposition of organic particles during the LGM, which is in line with observational evidence from sediment records. The nutrient inventory in the Mediterranean Sea is higher during the LGM compared to present day, but the simulated net primary production, i.e. biomass produced by photosysthesis after deduction of cellular respiration, is lower. Colder LGM temperatures reduce phytoplankton growth rates and increase the remineralisation length scale, which causes an increased export of organic matter towards greater ocean depth in the model. Furthermore, the maximum depth of the seasonal mixed layer is much shallower in the eastern Mediterranean Sea during the LGM than in present day, which leads to a decreased supply of nutrients to the biological productive surface layers. Our new model framework provides the basis to unravel the temporal and spatial drivers of the variability of signals found in sediment proxy records from the Mediterranean over the entire deglaciation.