Climate engineering?
"Geoengineering" or "climate engineering" may be defined as the deliberate large-scale manipulation of climate. The study of such techniques has been proposed in order to prepare for the cases that attempts to limit projected climate change by the reduction of greenhouse gas concentrations may fail or that consequences of climate change may prove worse than expected. Geoengineering may result in undesirable side effects for crucial parts of the Earth system and humankind. Therefore it is necessary to study the possible efficiency, risks and implications. Numerical models are the only possible tool for performing initial scientific studies. Complex climate models will be used to quantify the effectiveness and side effects of such geoengineering concepts aiming at a reduction of the incoming solar radiation.
In general two main classes of geoengineering techniques are distinguished:
Carbon dioxide removal techniques (CDR) address the root cause of climate change by removing CO2 from the atmosphere: e.g. technical capture of carbon from the atmosphere. ocean fertilization to enhance uptake by biological systems, increasing the alcalinity of the ocean or afforestation on land.
Solar Radiation Management techniques (SRM) reflect sun light and heat back into space. They attempt to offset effects of increased greenhouse gas concentrations by causing the Earth to absorb less solar radiation. We have performed computer simulations to study the climatic impact of different approaches:
- Enhancement of marine cloud reflectivity;
- Placing shields or deflectors in space to reduce the amount of solar energy reaching the Earth;
- Mimicking the effects of volcanic eruptions by injecting sulfate aerosols into the lower stratosphere.
- Manipulation of cirrus clouds
Stratospheric aerosol intervention (SAI)
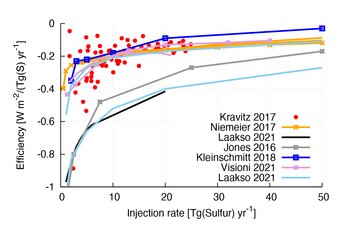
Our focus is on the evolution of sulfate in the stratosphere after an artifitial injection of SO2. We are interested in the size of the aerosols, the radiative forcing and the transport of the aerosols. The aerosols absorb light which heats the aerosol layer with impacts on stratospheric dynamics and transport of the aerosols. We wrote papers in the impacts on stratospheric dynamics, mainly the QBO (Franke et al, 2022; Niemeier et al 2017, 2020), climate (Niemeier et al, 2013).
The following text is a short version of a Science Perspective:
Research in this area gained traction after Crutzen’s call for investigating the effects of continuous stratospheric injections of sulfur into the atmosphere (Crutzen, 2007), as one method to deliberately mitigate anthropogenic global warming, analogue to observed lowering of temperatures after large volcanic eruptions. SAI could be seen as a last resort option to reduce the impact of climate change to society, e.g. increasing heat waves, floods, droughts, and sea-level rise.
SAI technologies are presently not developed, and scientists are just starting to grasp the potential risks and benefits of these kind of interventions. Various model studies have helped to improve the understanding of sulfur aerosol microphysical processes and transport of aerosols (e.g. GeoMIP), for example in realistically reproducing aerosol distributions after recent volcanic eruptions. A feature of aerosol microphysical processes related to SAI is the reduced cooling efficiency (cooling per injected unit sulfur) with increasing injection rate (see Figure). In short, the more SAI is done, the less effective further injections are at reducing temperatures. But, the amount of injection required for a given level of cooling is uncertain, varying from 0.1 to 0.4 Wm-2(Teragram(Sulfur) year-1) between different models, and also depends on injection location, -height, and -area.
Additionally, the resulting aerosol distribution patterns are uncertain and depend on the injection location and rate, and are also dependent on transport and chemistry in the models. Stratospheric sulfate alters the aerosol transport by warming the stratosphere due to absorption of terrestrial radiation. This warming impacts stratospheric dynamical processes, e.g. higher wind speed in the stratosphere at the equator decreases the poleward transport of aerosols (Niemeier and Schmidt, 2017). This has consequences not only for sulfate but for the transport of all stratospheric constituents. Changes in stratospheric chemistry have been shown to impact stratospheric ozone concentrations and cause a delay of the Antarctic ozone recovery by several decades (WMO O3 assessment 2022). Further links to our work: publications.